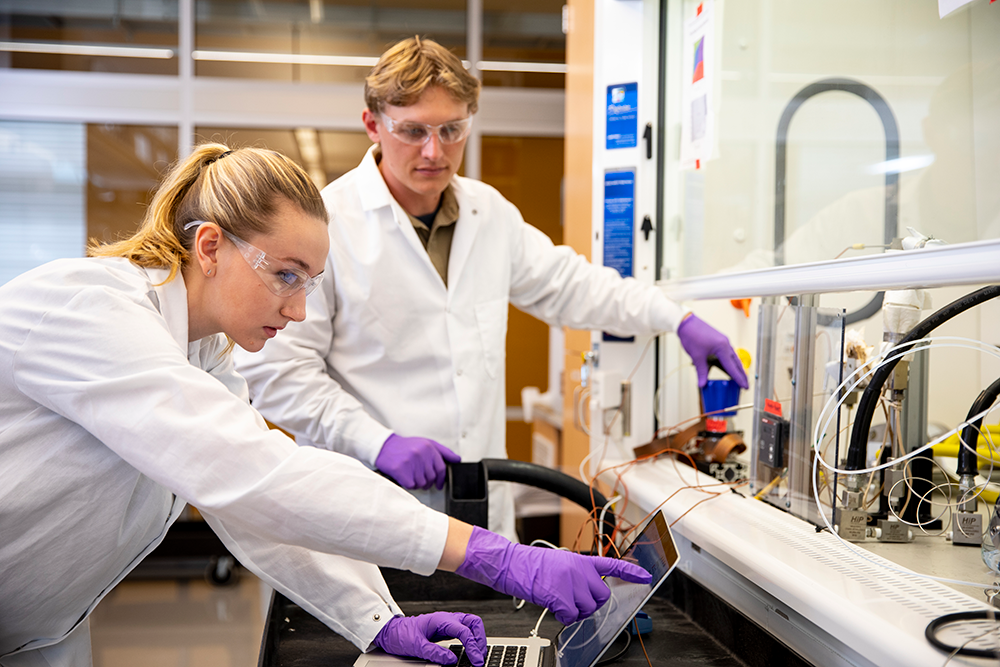
Metal-organic frameworks (MOFs), a unique engineered material first pioneered in the late 1990s, have long been touted for their potential versatility in next-generation climate and biotechnologies. Scientists have created nearly 100,000 different kinds of MOFs and have demonstrated their applicability in capturing and storing harmful gasses like CO2, removing toxic pollutants from water, serving as cathodes or anodes in batteries, and delivering drugs to specific targets in the human body. Many believe MOFs are poised to be the defining material of the 21st century. Still, they have yet to have a significant impact on society due to expensive, complicated, and time-consuming manufacturing processes.
A University of Washington research team has developed a new method to quickly, cheaply and sustainably synthesize large volumes of high-quality MOFs. In a recent publication in Chemical Engineering Journal, the team explored how changing various variables in their reactor would affect their ability to synthesize MOFs. They found that they could make high-quality MOFs under all conditions, demonstrating that their system is incredibly robust, a key factor in industry adoption.
“There are so many possible applications, our biggest challenge is figuring out where we can have the greatest impact,” said senior author Igor Novosselov, a UW associate research professor in mechanical engineering. “We are interested in finding partners, in academia or industry, that can help us identify the right application to move forward with commercializing this technology, connecting innovation with impact.”
MOF crystalline solids are composed of a structured network of nanopores that can be designed to trap specific molecules. This ability to be customized allows them to be used in many different applications. These sponge-like materials have an ultrahigh internal surface area enabling them to absorb enormous volumes of gases or liquids; however, they can only be produced in small quantities. Zeolites, a readily available natural material widely used in industry, are similarly porous to MOFs but are not very tunable or specific.
Typically, researchers make MOFs through a multistep process in which reagents are mixed, energy is added (usually in the form of heat), and then a cleaning process is activated to remove any reaction byproducts that may have gotten stuck in the nanopores. This chemistry is rather slow due to the diffusion limit between liquids and gases, making it difficult for molecules to find each other and form the necessary intermolecular contacts in the MOF structure.
“We wondered whether it would be possible to make MOFs using a continuous flow process in which everything is done at once,” said Novosselov, who is also a faculty researcher with the UW’s Institute for Nano-engineered Systems and Molecular Engineering & Sciences Institute. “The advantages of this approach are that diffusion is much faster, the process is more efficient, and it is easy to scale up because the necessary infrastructure already exists in certain industries like the petroleum industry.”
The Novosselov lab specializes in supercritical fluids – fluids pushed to a temperature and pressure where it is no longer possible to differentiate between the liquid and gas phase – using them to destroy organic substances or make nano- and micro- scopic particles. They have primarily experimented with supercritical water, which exists at pressures above 220 atmospheres and temperatures above 374 degrees Celsius. Such harsh conditions are not compatible with MOF synthesis, so the team turned to CO2, which goes supercritical at much milder conditions: 50 atmospheres of pressure and 31 degrees Celsius. Supercritical CO2 is also a well-known medium for dissolving organic solvents. Thus, supercritical CO2 does two things: it brings reagent molecules together faster and at the same time cleans the resulting product, removing any unwanted organic byproducts of the reaction and activating MOFs. Researchers have previously used supercritical CO2 to clean MOFs but never before has anyone combined these processes for single-step synthesis.
“This study would not have been possible without access to the UW’s Molecular Analysis Facility (MAF) or Washington Clean Energy Testbeds,” said Elizabeth Rasmussen, the study’s lead author who is now a postdoctoral researcher at the National Institute of Standards and Technology (NIST) in Boulder, Colorado. “I relied heavily on the equipment and staff expertise available at those facilities to thoroughly characterize an enormous number of experiments in a short period of time.”
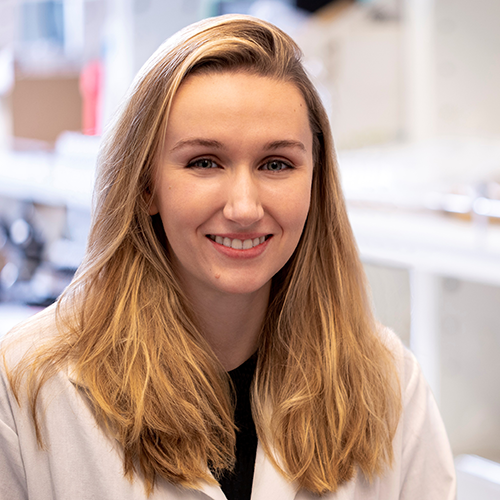
The MAF, an open-access facility located in UW’s Molecular Engineering & Sciences building, is part of the National Nanotechnology Coordinated Infrastructure, a National Science Foundation-funded effort to coordinate and support nanoscale research and development across the United States. The MAF offers microscopy, spectroscopy and surface science tools for the characterization and development of novel materials, advanced biomedical devices, photonic sensors and more. The Washington Clean Energy Testbeds offers customized training and use of instruments for fabricating prototypes, testing devices and modules, and integrating systems.
“As a mechanical engineer, my coursework did not include instruction on how to operate characterization equipment such as scanning electron microscopes (SEM), transmission electron microscopes (TEM), or x-ray diffraction instruments (XRD), but through the MAF, I was able to learn how to operate those instruments from experienced professionals,” said Rasmussen. “Not only were the skills I gained instrumental to my doctoral research, but they have proved incredibly valuable to advancing the research I’ve conducted outside of the UW.”
Now that they’ve demonstrated that their reactor works and is incredibly robust, Novosselov’s group is looking for collaborators to help apply this technology. While there are many potential applications, Novosselov is most excited by the opportunity to make MOF materials for separating two or more gases, such as the products of nuclear decay: Xenon and Crypton. Nuclear power plants and legacy waste sites experience ongoing emissions from spent fuel; MOF materials could capture these gasses before they are released into the atmosphere and allow them to either be stored or used elsewhere.
“While we look for partners interested in commercializing this technology, we are also investigating whether this system could be used to synthesize biological materials, carbon nanotubes, and composite materials,” said Novosselov. “The flexibility of our approach allows us to tune the properties of these materials, optimizing them for many different applications. MOFs are a remarkable material – it’s just a matter of time before we start seeing them everywhere.”
John Kramlich, a UW professor in mechanical engineering, is also a co-author. This research was partially funded by a grant from the US Defense Threat Reduction Agency. The team has also submitted two patent applications based on this work.
For more information, contact Novosselov at ivn@uw.edu.
—
Synthesis of metal-organic framework HKUST-1 via tunable continuous flow supercritical carbon dioxide reactor.
Elizabeth G. Rasmussen, John Kramlich and Igor V. Novosselov
Chemical Engineering Journal
DOI: 10.1016/j.cej.2022.138053